Cell culture: ask the experts
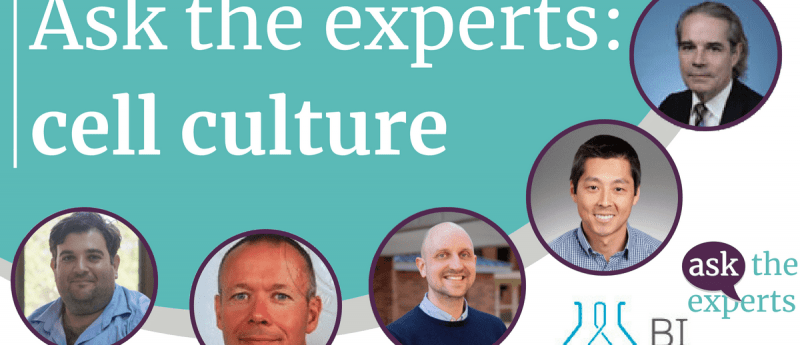
In this ‘Ask the Experts’ feature, a panel of international experts share their perspective on current obstacles and future developments in cell culture.

Cell culture is a vital step in the development, manufacture and commercialization of any cell-based therapy. Addressing and monitoring your cell culture will support more consistent, stable and effective products.
In this ‘Ask the Experts’ feature, we’ve collected insights from experts around the world to discuss the development of cell culture and what still needs to be done.

Jump to:
- How have cell culture needs changed since you began working in this field?
- How can cell culture protocols be adapted to meet the different needs of different applications?
- With the important role that cell culture plays in the approval process of cell therapy, how can developers simplify this process?
- What is the biggest obstacle remaining in the field?
- What’s the best/most effective technology innovation you’ve seen in the last 5 years?
- How do you see cell culture processes, protocols and products evolving in the next 10 years?
How have cell culture needs changed since you began working in this field?
Oren Ben-Yosef (OBY): It depends who you ask, I think. Researchers and labs will tell you that the needs are the same — good looking cells, or colonies, that expand indefinitely and differentiate to whatever somatic cell or cells they are researching. The industry, the pharma companies and everybody who is doing clinical applications will tell you that the needs have changed drastically; more regulatory requirements call for more defined culture conditions and the cells must be expanded better. Time is money and a shorter culture time to get the same amount of cells, or more cells, saves money.
Jorge S. Burns (JSB): When I began working in the field it was a genuinely in vitro world. To address needs of convenience and safety, this was replaced by disposable plastic cell culture. Over the last three decades, increased sophistication has greatly improved primary human cell culture. Lessons learned from immortal human cancer cells are now being tailored for a much wider range of cell and tissue types. This in turn has revolutionized our understanding of pathological processes in many previously untreatable diseases. In particular, appreciation that tissue specific stem cells may serve as therapeutic agents has driven huge expansion and progress across academic, industrial and clinical sectors. Multidisciplinary collaboration is now fundamental.
Justin Colacino (JC): The field has changed dramatically since I first started doing cell culture back in 2005. There has been an explosion of methods to grow patient derived tissues in three dimensions. We can now generate induced pluripotent stem cells (iPSCs) from anyone, and then use directed differentiation in a dish to push these cells into an expanding array of cell types. Advances in microfabrication now allow us to better mimic the physical environment within a tissue, and even model how multiple tissues communicate. On top of this, agencies such as the US Environmental Protection Agency and the National Institutes of Health are making concerted efforts to replace animal experiments with well-designed and validated in vitro experiments. In short, it’s an exciting time to be doing cell culture.
Daniel Kota (DK):There has been a significant increase in demand for consumables, a proliferation of good manufacturing practice (GMP) facilities and multiple attempts to solve large scale manufacturing. This has all been triggered by the ‘discovery’ of stem cells, such mesenchymal stem/stromal cells (MSCs), embryonic stem cells (ESs), iPSCs, along with tissue-specific stem cells including neural or adipose cells, combined with the most recent excitement caused by CAR T, NK cells, and dendritic cells for immunotherapy applications.
These cells are being used in both basic and clinical research, at small and large manufacturing scale worldwide. Hence, it’s not unusual to experience backorders of nutrient media like alpha MEM and plasticware. For instance, the wait for Corning’s Hyperstack technology used for cell expansion can reach over 1 year, although the company has announced efforts to increase capacity. In addition, the price of serum has seen an increase in 1000% for the past 10 years. Human platelet lysates and chemically defined media, although increasing in availability, offer the same high prices.
There is also a need for a hybrid workforce that combines cell culture, regulatory and clinical knowledge that neither laboratory technicians nor medical technologists alone offer, and there is still no consensus on the optimal bioreactor or large scale technology for a lot of cell types, including MSCs.
Bruno Péault (BP): I cannot say that our actual needs have much changed. Generally speaking, we culture cells either to “expand” them, that is to collect larger numbers thereof (and this is the main goal of cell culture for clinical applications), or to study their biology in a relatively simple setting. In the field of stem cell research, for instance, a number of differentiation potentials can be readily assessed in vitro: turning stem cells into bone, fat, cardiac muscle or some blood cells in culture is relatively easy, for instance. Even though the cells we are studying have diversified dramatically, what we expect from cell culture remains essentially the same.
There are, however, some evolutions, such as cell culture under different oxygen concentrations, which has literally exploded in recent years. In the atmosphere, therefore in standard cell culture conditions, oxygen is present for 20%. It has been discovered that culturing cells in lower oxygen concentrations, such as 5% and even 2%, changed dramatically their behavior and properties. The reason is that genes encoding proteins named hypoxia inducible factors (HIFs) are activated under lower oxygen concentration (hypoxia). Changing cell properties under hypoxia is not a mere culture artefact though, but reflects the fact that, depending on the extent of vascularization, some tissues in the body are naturally hypoxic. A whole area of pathobiologic research tries to understand how oxygen concentration regulates cell function, which is of high relevance in organ development and regeneration, as well as cancer.
In another perspective, cell culture is increasingly combined with biomaterial science to determine optimal cell survival and growth conditions, in two and three dimensions. To this aim, cells are cultivated in the presence of adhesion molecules, synthetic matrices and scaffolds. Beyond basic biology, this research aims to determine optimal “packaging” conditions for therapeutic cells to be transplanted into patients.
How can cell culture protocols be adapted to meet the different needs of different applications?
OBY: I think adaptation happens all the time — new media are being developed, existing media are modified and new matrices are discovered. I believe that it is not the cell culture protocols that need to adapt, it is the researchers themselves. The one dichotomy I always observe when visiting labs is that scientists, while being as broadminded as they should, as they must, can be very, very narrow-minded, even having “tunnel vision”, when it comes to their protocols. I often hear: “It has been working for 20 years and I’m not changing anything!” Things have changed, radically, in the last 20 years…as with everything in life, you must adapt, or die — metaphorically, at least!
Therefore at Biological Industries we try to let researchers know that protocols are not sacrosanct, and that how they grow their hPSCs does have an effect on their differentiation and experiments — it is well known now that high levels of bFGF, for instance, inhibit differentiation patterns. So we try to suggest to them to switch to media that is low in growth factors concentrations. Certainly protocols that were published 10-20 years ago can be a good base for tweaking.
JSB: Protocol adaptation is key to obtaining cellular performance that more relevantly represents physiological behavior. A trend from 2D to 3D culture arguably helps improve mimicry of in vivo conditions as does bioreactor development towards more dynamic cell maintenance conditions.
JC: There is a dizzying array of tissue culture protocols and methods available. In our lab, we’re adapting techniques for the 3D culture of precancerous tissues from human breast and colon. These experiments will typically involve altering the composition of the media or assessing different growth matrices. We often also modify our culture conditions to enrich for cell types within a culture, for example, using conditions that enrich specifically for stem cells. More recently, we have been interested in adapting our experiments to medium- or high-throughput screens, which have led us to modifying our cultures to work in a smaller volume in ways that are amenable to high content image analysis.
DK: Cell culture protocols have to adapt to balance two very important aspects: growth versus function. In particularly for stem cells, rapid growth does not guarantee enhanced functionality. In fact, it may have the opposite effect. While this is more easily measured in cell types in which differentiation itself probably holds the therapeutic value, like iPSCs and ESs, for cells like MSCs, however, the lack of defined mechanism of action despite decades of research and many potential candidates, poses a critical challenge. We might, in fact, be measuring the wrong or insufficient parameters to determine therapeutic efficacy.
As for immunotherapies, I believe there is need for more studies assessing how manufacturing under extensive proliferation or different culture conditions may affect function and side effects like the cytokine storm. One could speculate that a rapid proliferation of immune cells signals danger, which in turn might predispose the same immune cells to be more responsive and inflammatory in nature.
Jump to:
- How have cell culture needs changed since you began working in this field?
- How can cell culture protocols be adapted to meet the different needs of different applications?
- What is the biggest obstacle remaining in the field?
- What’s the best/most effective technology innovation you’ve seen in the last 5 years?
- How do you see cell culture processes, protocols and products evolving in the next 10 years?
With the important role that cell culture plays in the approval process of cell therapy, how can developers simplify this process?
OBY: It’s important to work from the ground up, have a good regulatory team in place, including QA and RA, and obviously work with the highest order suppliers, so all the raw materials are defined, known and approved. For instance, BI has media that have a Drug Master File (DMF),submitted to the US FDA and Health Canada, that contains detailed information about the ingredients, manufacturing facility and procedures involved in the production of a specific product. The process of registering a new cell therapy product with the FDA is significantly shortened when utilizing a product with a DMF. It also demonstrates to the consumer that a company that manufactures such a product conducts its manufacturing operations in a transparent and correct manner and complies with quality and safety requirements.
One place that that took advantage of this is the Ottowa Hospital Research Institute (ON, Canada) that has a clinical trial called “Cellular Immunotherapy for Septic Shock” which is the result of research led by Dr. Duncan Stewart and Dr. Lauralyn McIntyre. While MSCs have been used in clinical trials for other conditions, this is the first trial in the world to see how the cells specifically treat septic shock. In one case, a septic patient was injected with 30 million MSCs, which researchers believe may tamp down severe inflammation, based on animal studies that showed they can overcome sepsis and significantly reduce mortality. After three months in the ICU and another month or so recovering at home, he was restored to health and was able to return to normal life.
JSB: Simplification is key to broad adoption of cell culture conditions and practical transferability from academic to clinical scenarios in a cost-effective manner. At the same time, simplification mustn’t undermine appropriate mimicry of in vivo conditions. Compromises are perhaps inevitable, but advances in microfluidics, new material nanotechnology and improved biomarker discoveries are conspiring to increase the choices available for changing and controlling cell micro environments. Despite many challenges, those novel options allow an optimistic outlook.
JC: An area where cell culture product developers can have a significant impact in the rigor and reproducibility of the field is in developing media and other culture components which are fully defined. Many of the products we use in our work are biologically derived, and thus susceptible to significant lot-to-lot, and thus experiment-to-experiment, variation. By developing fully defined cell culture reagents, product developers can help reduce this variation and improve reproducibility across multiple laboratories.
DK: Developers have already begun and must continue to work with manufacturing sites on personalized media that come ready-to-use. The reduction of reagent amalgamation and media changes that cell processing facilities have to perform not only decreases contamination risks and human error, but also alleviates the regulatory burden. It should be noted that the latter should always be in mind when developing any cell culture reagent.
BP: Culture complicates approval by regulatory agencies. The culture process is generally rather simple and highly standardized though. I guess cell cultivators just have to bear with regulations; I don’t really see many ways around.
However, it is sometimes possible and more original to simply bypass the culture step. This is one of our strategies, using selected native “unexpanded” stem cells. Contrary to conventional thinking, you do not necessarily need to “expand” stem cells in culture prior to therapeutic use. If you have the right stem cells you do not need lots of them, although this does not apply in all instances. You remain of course dependent on culture if you are using pluripotent cells, such as ESs, iPSCs, or conventional MSCs, which are in essence selected by the culture process.
Unexpanded stem cells are purified from dissociated tissues by fluorescence activated cell sorting. The whole process takes a few hours, during which the cells are maintained at a low temperature in sterile balanced salt solutions supplemented with fetal calf serum, which guarantees high viability and minimal if any changes in biologic characteristics.
What is the biggest obstacle remaining in the field?
OBY: This relates to the previous question. The biggest obstacle, but a necessary one, is the regulations that are in place for cellular research, and clinical research in particular. Regulations are placed for a reason, and a good one at that. They might hinder some projects, but if your research is robust enough, and if you have a good support from your suppliers, it becomes less of an obstacle and more a hurdle that shows that you are well on the way to achieve what you want — good, consistent research that can benefit mankind.
It is important to note that as a manufacturer for the life science industry, BI is fully committed to the current Good Manufacturing Practices (cGMP) comprising applicable local law as well as international guidelines adopted by the FDA as well as the European Medicines Agency (EMA). BI’s quality systems comply with the international standards for Quality Management Systems as defined by the ISO 13485:2016 and routinely audited by an accredited external body. Our manufacturing facility is an ISO 9001:2015 and ISO 13485:2016 certified facility and our controlled environment clean rooms are graded from ISO 8 up to ISO 5 (classified from class 100,000 to 100). We offer all of our expertise and facilities for our customers to take advantage of and use.
JSB: Obstacles remaining in the field are many. An understandable focus on isolation and maintenance of pure cell cultures, often expanded as monolayers, is being superseded by a growing need to understand cell-cell interactions between different cell types, also within organoid tissue-like contexts. Vascular development, cell homing, extracellular vesicle mediated intercellular communication and appreciation of mitochondrial homeostasis are areas of research that will require complex analysis. Fortunately, progress in instrumentation and technological know-how including label-free and single-cell analysis will facilitate steady progress. Ultimately, accurate measurements and identification of key active components will lead to approved potency assays that are critical for designating the quality of a cell product for therapeutic use.
JC: There are a number of important challenges still facing the in vitro field. I think the biggest obstacle that we currently are facing is how to accurately model the full diversity of cell types within a tissue and their interactions. In our lab, our cultures typically have, at most, two to three different major cell types present. We know, especially in cancer, that the stromal fraction of the microenvironment plays a huge role. Capturing these interactions in a physiologically relevant way remains a major challenge for us.
DK: The biggest challenge remains the translation from bench to bedside. It’s not possible for a research lab to undertake the costs of large scale manufacturing. Therefore, most of it is done offsite or by private companies and ended up ‘lost in translation’ simply because the nuances and conditions seen in the laboratory cannot be fully replicated. Many basic studies are performed with fetal bovine serum (FBS), which is not used in patients. The use of the patient’s plasma, platelet lysate or chemically-defined media may never replicate the specific characteristics observed on the bench or animal models. Likewise, the use of bioreactors may alter cells that were originally cultured in flasks.
We know that shear stress can change the properties of MSCs when compared to static cultures [1], along with doubling time and confluence. Again, it comes down to define mechanisms, but also foresee possible complications and side effects that may originate from large scale that are often overlooked.
BP: I would not call it an obstacle but a complication, a bias which is too often overlooked. The fact is that culture dramatically changes gene expression in cells. Some, like MSCs, have long been characterized exclusively as cultured cells. Now that we know what innate MSCs are within tissues, we can make a direct comparison and we have realized that MSCs are dramatically modified by culture. MSCs are the most commonly used human stem cells in the clinic, with more than 800 trials to date. Many users assume that cultured MSCs are exact replicas of native, tissue resident MSCs, which is wrong. Quantitatively, as well as qualitatively, MSCs are largely a product of in vitro culture. This is of course likely applicable to other cultured cells. This is why culture assays often cannot replace in vivo experiments.
What’s the best/most effective technology innovation you’ve seen in the last 5 years?
OBY: It’s not a “technology” per se, but I think that, finally, researchers have started looking more at the macro rather than the micro. Organoids, one of the “hottest” research methods of the last years, are 3D in vitro grown structures derived from pluripotent stem cells (PSCs) or adult stem cells that self-organize into near-native microanatomy with organ-specific differentiated cell types and tissue compartmentalization. The reason I think this is the most effective technology is that you can’t take a cell population out of context, for example it’s native tissue or organ, and expect it to behave as it does in vivo or in situ. Therefore creating the microenvironment of the organoid is far more indicative to PSC research than any changes in incubators.
JSB: Many technological innovations emerging in the last five years have a long history in the making. What is most significant is that cells have been approved as therapeutic agents and supporting technologies have become more refined. Significant advances in imaging technology have improved diagnosis and our ability to track therapeutically applied cells. Use of theranostics when monitoring new therapeutic interventions will help accelerate clinical trials. If pressed to describe the best/most effective technological innovation in the last 5 years, then advances in immunology are clearly outstanding.
JC: While it’s not an innovation that is specific to cell culture, the ability to comprehensively profile large population of single cells using unbiased analyses is, I believe, pushing our field forward more than any other. In our own work, we are using single cell RNA-sequencing to map our primary human cultures and have uncovered new and exciting biology to pursue. These studies are informing our in vitro work by giving us a comprehensive catalog of the cell types and phenotypes in our cultures. We can now understand in exquisite detail the effects of altering media composition or exposing the cells to an environmental stressor, on a cell type specific basis.
DK: Any technology that automates cell isolation, such as Sepax Technologies, Inc. (DE, USA), processing, assessment, for example cell counters or benchtop flow cytometry, or storage, such as CellSeal from Cook Regentec (IN, USA), are improved technologies.
BP: I may have missed something but in our areas of investigation I am not aware of any major recent innovation as regards cell culture. At least with regard to basic research, cell culture has changed amazingly little. If we go back 25 years, for instance, we used the same incubators, the same plastic ware, the same media and the same antibiotics, and we were equally dependent on the use of animal serum. If you compare with the evolution of molecular biology techniques, for example, the difference is absolutely striking.
How do you see cell culture processes, protocols and products evolving in the next 10 years?
OBY: In one word? Suspension.
Clinical applications require more and more cells to be effective. While refinements to differentiation protocols are done all the time, the more cells you have, the more end product — be it other cell types, secreted proteins, vaccines or whatnot — you get. There is a lot of work being done to adapt PSC and mesenchymal stem cell culture to 3D culture conditions, which include better suited media, microcarriers and/or hydrogels, organoid and aggregates formation, better suited bioreactors and many other advances happening right now, all with the end goal of getting more cells each passage while maintaining their characteristics and markers.
JSB: Cell culture processes, protocols and products are going to be increasingly influenced by information and communication technology and the elephant in the room is how data handling will need to be controlled and regulated within increasingly knowledge-based economies. Regulations regarding privacy of information will impinge upon laboratory activities as operating procedures become increasingly standardized.
Accordingly there will be a period requiring increased investment in robust biomarker discovery and biosensor design to improve monitoring and quality control. However, key to implementation will be focus on efficiency, sustainability and cost reductions for broad use. Over the coming years bioreactors and perhaps implementation of robotics guided by artificial intelligence may become key elements of industrial scale processes that bring cell-based therapeutics to a demographically growing market. Novel products that specifically activate and regulate innate stem cells for improved healing are also likely to emerge.
JC: I expect that the next 10 years will see an explosion in the number of available patient derived organoids in biobanks. I hope that these tissues will be collected from epidemiologically characterized individuals who, in aggregate, better represent human genetic diversity. I expect that these organoids will allow us to make significant progress in truly understanding the biology of gene-environment or gene-pharmaceutical interactions. I also expect that we’ll see significant improvement in 3D models of the tissue microenvironment through improved culture protocols, medias and matrix development.
Finally, I suspect that we’ll see dramatic democratization of the “organ on a chip” methods through increased access to microfabrication facilities and 3D printers. Compared to the organoid models our lab typically uses, the culture conditions for “organs on a chip” are complex, as they require culture conditions that are simultaneously suitable for multiple cell/tissue types. These conditions can range from differences in growth matrix, to media formulation, to oxygen concentration. As we overcome these challenges, however, we will be closer than ever to accurately modeling human physiology in a tissue culture dish.
DK: In the next 10 years, it is safe to say every major hospital will have its own cell manufacturing facility since cell therapy will be extremely personalized and the technology to manufacture cells will be available to all. We will see culture media that are personalized for each cell application, with ready-to-use, off-the-shelf products that will minimize preparation and media change. Finally, automated, closed systems will become more available and the current need for trained workforce will be alleviated.
BP: It is hoped that evolution will be seen in 3D culture systems to mimic more closely tissue structure. On the other hand, a major challenge has been, for decades, to maintain “natural” stem cells such as hematopoietic stem cells in an undifferentiated state in vitro. Progress in this direction would represent a major breakthrough.
References:
1. Diaz MF, Vaidya AB, Evans SM et al. Biomechanical Forces Promote Immune Regulatory Function of Bone Marrow Mesenchymal Stromal Cells. Stem Cells. 35(5): p. 1259-1272 (2017)
Jump to:
- How have cell culture needs changed since you began working in this field?
- How can cell culture protocols be adapted to meet the different needs of different applications?
- With the important role that cell culture plays in the approval process of cell therapy, how can developers simplify this process?
Meet the experts
Oren Ben-Yosef, PhD (Technical Support, BI Israel)
Oren Ben-Yosef joined Biological Industries in 2016 as a Technical Support Specialist. Oren has an BSc in Biotechnology Engineering and a PhD in Medical Sciences from the Technion Israel Institute of Technology. He conducted his research in the laboratories of Prof. Ido Perlman and Prof. Joseph Itskovitz-Eldor, focusing on embryonic stem cells and induced pluripotent stem cells culturing methods and differentiation.
Jorge S. Burns (University Politehnica of Bucharest)
Jorge S. Burns is Project Coordinator for the GRABTOP Project which aims to develop a novel graphene biosensor testing osteogenic potency for capturing best stem cell performance for regenerative medicine. He is part of the Faculty of Medical Engineering at University Politehnica of Bucharest (Romania).
Justin Colacino (University of Michigan)
Justin Colacino is the John G. Searle Assistant Professor of Environmental Health Sciences in the School of Public Health, University of Michigan (MI, USA). His research focuses on understanding environmental and dietary factors in carcinogenesis and cancer prevention. Specifically, the goal of his research is to characterize the environmental susceptibility of normal human stem cell populations, elucidating the etiology of sporadic cancers. His research group combines wet lab bench work, and bioinforatic and statistical analysis of large scale genomic and epidemiologic data sets to translate findings from in vitro models to the population level. Dr. Colacino is an active member of the Society of Toxicology (SOT) and the American Association for Cancer Research (AACR) and serves on the Editorial Review Board of Environmental Epigenetics and the Editorial Boards of Cancer Research Toxicological Sciences and Epigenomes.
Daniel J. Kota (Emory University)
Dr. Daniel J. Kota is an Associate Scientist and Director of Emory Personalized Immunotherapy Core (GA USA). Native of Brazil Dr. Kota received his PhD in medical sciences completed a postdoctoral training in neurotrauma and ran a research program as faculty unlocking the therapeutic properties of adult stem cells across the USA at the Burnham Institute (CA USA) Tulane University (LA USA) Texas A&M University the University of Texas (both TX USA) and Sanford Health (SD, USA).
Bruno Péault (University of California at Los Angeles)
Bruno Péault is Professor and Chair at the University of Edinburgh (UK) Center for Vascular Science and MRC Center for Regenerative Medicine and Professor of Orthopedic Surgery at the University of California at Los Angeles (UCLA). Dr Péault’s research includes developmental hematopoiesis as well the identification purification and characterization of diverse classes of stem cells present in adult organs. Prior to joining UCLA and the University of Edinburgh Péault served as a Professor of Pediatrics and co-director of the Stem Cell Research Center at the Children’s Hospital of Pittsburgh (PA USA) as research director at the Centre National de la Recherche Scientifique and department head at the Institut National de la Santé et de la Recherche Médicale in Paris.