Cellular reprogramming to model the human brain in vitro: an interview with Cedric Bardy
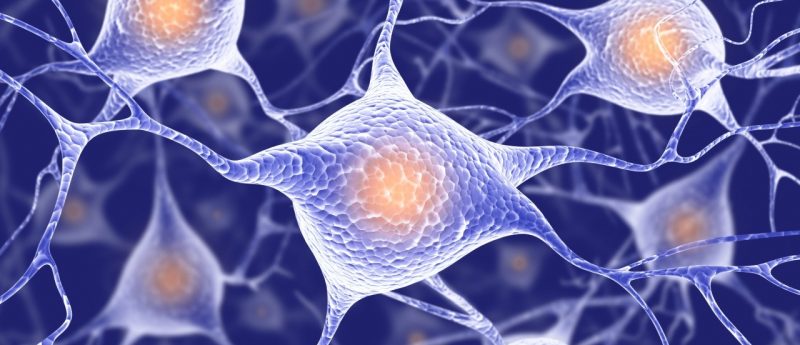
In this interview, Cedric Bardy, the Director of the Laboratory for Human Neurophysiology and Genetics at SAHMRI Mind & Brain in Australia, discusses progresses in stem cell reprogramming technologies that have the potential to lead to the discovery of new clinical treatments for neurological and psychiatric disorders.
Cedric Bardy
Dr Bardy is an expert in human neural stem cell models, electrophysiology and single-cell genetics. His research efforts are focused on developing new stem cell biotechnologies and strategies to understand the effect of neurological disorders on brain cells. He obtained a PhD in Medicine from the University of Sydney (Australia) in Dr Bogdan Dreher’s Laboratory (2004—2008). He followed his postdoctoral training in Neuroscience in the laboratory of Dr Pierre-Marie Lledo at the Pasteur Institute in Paris (2008—2010). He then received the prestigious Marie Curie international fellowship to pursue his research in the laboratory of Dr Fred Gage at the Salk Institute in California (2011—2016). In 2016, he was appointed Assistant Professor/Senior research fellow at the newly established South Australian Health and Medical Research Institute, and Senior Lecturer at the School of Medicine of Flinders University.
Website: www.bardylab.com
Email: [email protected]
Could you tell us a little about your career to date and what drew you into the field of neuroscience?
The complexity and power of the human brain fascinates me. I spent many years as a junior researcher scrutinizing how neural circuits process sensory inputs to create coherent visual perception, olfaction, movement and memories. It’s incredible that even the most powerful computers do not compete with the ordinary tasks that the human brain can perform. The brain is also beautifully designed to adapt to a wide range of changing biological conditions.
However, in some instances such brain plasticity reaches its limits and fails to maintain a balanced healthy neuronal activity. The consequences are serious and manifest in a variety of debilitating neurological disorders such as Parkinson’s disease, Alzheimer’s disease or depression. My lab is investigating the possibilities that some individuals have specific genetic predispositions that make it harder to cope with biological stressors and maintain a balanced brain activity. Understanding these molecular mechanisms at the cellular level will provide solid basis to discover medical alternative that will help patients coping with serious brain disorders.
Why is stem cell research important for your work?
To increase our chances of discovering new efficient medical treatments, we must diversify experimental models. Human cell reprogramming technologies have rapidly become a vital element in the medical researchers’ toolkit, especially in the field of neuroscience, where access to human brain biopsies is limited. The potential to unlimited access of live human neuronal tissue in vitro with stem cell technologies give new hopes for better understanding of neurological disorders.
“Human cell reprogramming technologies have rapidly become a vital element in the medical researchers’ toolkit, especially in the field of neuroscience, where access to human brain biopsies is limited.”
What does your current role entail?
I have been fortunate to join the pioneering lab of Professor Fred Gage at the Salk Institute (CA, USA) at an exciting time, when iPSC technology breakthrough unlocked new, wide research possibilities. I also quickly realized that despite the hype and hope in the neural stem cell field, the current in vitro models were quite distant from basic neurophysiological conditions in vivo.
To really take advantage of the full capability of powerful neural in vitro models, tissue culture conditions need to be optimized. Reducing the artificial gap between in vitro and in vivo models is essential because more physiological models relevant to human diseases will increase our chances of translational success. There is a pressing societal need to discover new treatments for neurological and psychiatric disorders: my lab aims to improve research biotechnologies and directly apply them to tackle brain disorders such as Parkinson’s disease and major depression. Our main objective is to better understand the complex biology of the human neurons in the context of mental health and neurological disorders.
“Reducing the artificial gap between in vitro and in vivo models is essential because more physiological models relevant to human diseases will increase our chances of translational success.”
Do you think that reprogramming with non-DNA methodologies is the future, owing to the reduced risk of genetic mutations and abnormal expression compared with methods using viruses and vectors?
Cellular reprogramming and expansion in vitro may introduce undesired genetic mutations. Such artificial mutations may bias analysis in disease models or add hazards for cell transplantation therapies. This risk may be significantly reduced by using a non-integrating viral vector (e.g., Sendai virus) or non-DNA methodologies to reprogram cells into iPSCs. In addition, particular care should also be drawn towards mutations that might occur spontaneously, in particular during cell divisions [1].
What are the main non-DNA methods currently being investigated or developed for generating neurons?
The original successes of cell reprogramming were achieved with viral vector that helps integrating specific sequences in the DNA of the host cells to activate a set of transcription factors. Many alternative or complementary methods such as chemical agents, mRNA transcripts, or proteins are also being investigated [2].
If proven efficient, an interesting aspect of small-molecule reprogramming is that it may provide cell biologists with a more precise control: specific combinations and concentrations may be used to differentiate various types of neurons. The current goal in the field is to establish a large repertoire of methods to differentiate cells into the exceptional diversity of brain cells.
Are small-molecule tools the future of cell reprogramming?
Viral vectors and transgenes are often considered as a safety risks for human therapy [3]. ‘Small-molecules-only’ reprogramming and differentiation may prove safer. In addition, small molecules are more practical to manipulate. To become the gold standard in the field, small-molecule reprogramming protocols will have to be scalable, cost-effective, reliable (low variability), and most importantly very effective in term of reprogramming success.
Does direct conversion to generate neural cells look promising?
Direct conversion methods skipping embryonic developmental stages are complementary to iPSC-derived models. Pros and cons have been discussed at length over the last few years [1]. The main advantage of direct conversion is probably the simplicity of the protocols and the shorter time required to obtain neurons. However, the time in vitro to obtain functionally mature neurons appear highly variable both with direct and iPSC neural differentiations. Reaching functional maturity is often an important requirement for disease modeling and need to be rigorously controlled to take full advantage of these models [4].
Are there any different types of neuronal cells that are more difficult to culture and/or reprogram than others?
The human brain is a complex tissue with exceptional cellular diversity. Currently, available protocols focus on generating the major neurotransmitter classes of neurons such as glutamatergic, gabaergic, dopaminergic and serotoninergic. Interestingly, the identity and function of these neurotransmitter classes may diverge depending of the brain region analyzed in vivo.
More sophisticated protocols aim to push the identity to subcategories, like for example specific kind of dopaminergic neurons from a specific brain region. Furthermore, many other non-neuronal brain cells like glia, astrocytes (extremely diverse in humans), microglia and oligodendrocytes are critical for healthy brain functions. Rigorous protocols generating a range non-neuronal brain cell subtypes are also essential to create physiological neural circuits models in vitro.
You developed a cell culture medium that better supports neuronal activity, BrainPhys [5], by comparing the electrophysiological activity of human stem cell-derived neurons in different media and identifying the supporting and suppressive components. How long did this process take, and were any results particularly surprising?
I believe this process took much longer than it should have, probably because we were reluctant to question the validity of classical basal media that have been used somehow successfully for decades. The results were extremely surprising. It took a lot of hard scientific evidence to admit that the most fundamental functions of neurons, which are firing action potential and synaptic communication, were strongly un-physiological in routinely used classic media.
“It took a lot of hard scientific evidence to admit that the most fundamental functions of neurons, which are firing action potential and synaptic communication, were strongly un-physiological in routinely used classic media.”
What is the greatest challenge in accurately reconstructing the in vivo extracellular environment of neuronal cells?
For me, the main challenge was that these environments contain a very high number of components. If you multiply these by different concentrations, the possible combinations are huge. The gold-standard electrophysiological method, patch-clamping, which we used to assess the functionality of the neurons, is too low throughput to test them all. Therefore, we could not be completely agnostic in our testing and had to make some guesses based on current knowledge.
What would the next steps be to refine the neuronal media or culture process even further?
With BrainPhys, we tried to address the biggest issues that were in the basal medium. Now refinement steps will take significantly more work but are also necessary. It is a balance between mimicking in vivo conditions and a pragmatic approach of what works with the cells in vitro.
Looking forward, what do you predict will be the biggest hurdles to overcome for reprogrammed cells to achieve widespread clinical application?
I think variability and reproducibility in vitro is the biggest challenge. This is a challenge that must be addressed both for disease modeling in vitro and cell therapy applications.
What do you hope to have achieved in 5—10 years?
The field of neural stem cell is moving really fast and I am excited for the opportunity to contribute to its growth. Imagining the progresses that the entire community of researchers will have made in 10 years is exhilarating. We still have a lot of challenges ahead to consolidate these powerful medical research models.
Personally, I hope to be able contribute significantly to make the model more physiological, and to have the opportunity to take one of the fundamental discoveries made in our lab to the next step of clinical translation.
Acknowledgements
We acknowledge the support of Mr Lang Walker and Flinders University.
Financial & competing interests disclosure
No competing interests.
References
- Mertens J, Marchetto MC, Bardy C, Gage FH. Evaluating cell reprogramming, differentiation and conversion technologies in neuroscience. Nat. Rev. Neurosci. 17(7), 424-437 (2016)
- Hu W, Qiu B, Guan W et al. Direct conversion of normal and Alzheimer’s disease human fibroblasts into neuronal cells by small molecules. Cell Stem Cell 17(2), 204—212 (2015).
- Thomas CE, Ehrhardt A, Kay MA. Progress and problems with the use of viral vectors for gene therapy. Nat. Rev. Genet. 4(5), 346—358 (2003).
- Bardy C, Van den Hurk M, Kakaradov B et al. Predicting the functional states of human iPSC neurons with single-cell RNAseq and electrophysiology. Mol. Psychiatry (2016) (In press).
- Bardy C, van den Hurk M, Eames T et al. Neuronal medium that supports basic synaptic functions and activity of human neurons in vitro. Proc. Natl Acad. Sci. USA 112(20), e2725—e2734 (2015).