Cutting out the middleman with direct cell conversion: an interview with Rodrigo Santos
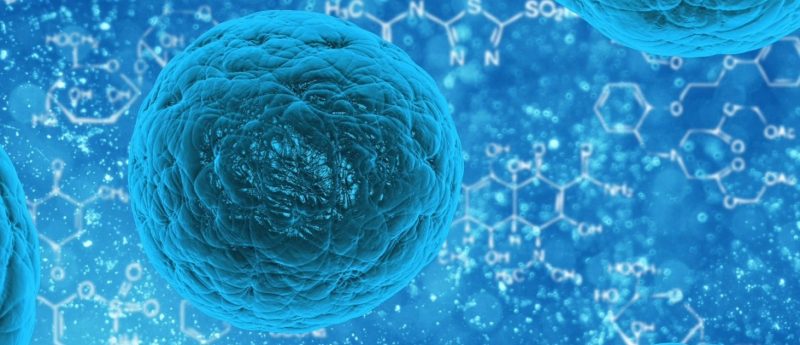
In this interview, part of the RegMedNet Spotlight on iPSC differentiation and expansion, Rodrigo Santos (Mogrify, Cambridge, UK) explains more about Mogrify’s big data-based direct cell conversion for cell-based therapy.
Could you introduce yourself and Mogrify?
At Mogrify, we are combining both reprogramming, where we can convert one cell into the other, and genome engineering, either by adding genes or removing genes, making use of these technologies to create novel cell therapies using direct cell conversion.
I have been at Mogrify for one year; prior to Mogrify, I worked for four years in genome engineering at a company called Horizon Discovery, where I trained as a genome engineer. Prior to that, I did my PhD in iPSC biology at the Stem Cell Institute in Cambridge, which I joined in 2011, where I was fortunate to join the laboratory of Jose Silva to look at the mechanisms underlying the generation of induced pluripotent stem cells (iPSCs).
What are the current challenges with converting cell types for therapeutic purposes?
The challenges associated with cell therapy can broadly be categorized into three key pillars; safety, efficacy, and scalability, which Mogrify aims to address with its proprietary direct cellular conversion technology.
Why safety? Now, when one thinks of cell therapies, you can pretty much divide them into immuno-oncology and regenerative medicine. Immuno-oncology involves more short-term cell therapies, where you need the cells to act for a short period of time. On the other side, on regenerative medicine, we are looking for long-term engraftment, for example, neurons to treat Parkinson’s or to effect cardiac myocyte regeneration in the heart.
Especially in terms of regenerative medicine, safety is paramount because you need to make sure that what you are introducing is beneficial in both the short-term and the long-term. For example, you must look at the stability of cells and their potential to become cancer cells. In terms of stem cell-derived therapies, we are concerned with the rate of conversion; if the conversion is not complete, how do we get rid of the cells that did not convert, and what are they? Are they progenitors, which could become somewhere else, or are they terminal?
The second pillar is efficacy. Are we sure that the cells that we are making are functional? Do they have bioequivalence? Are they behaving the same as the cells that would exist in that same tissue in vivo?
Let us look at diabetes: do we need to make cells that are exactly the same as beta cells or do we need to make cells that are functional at making insulin after response to glucose? There is no right answer. It is quite a divided field, but in the end, they need to be efficacious. If they do not produce insulin in response to glucose when they are in the body, regardless of how they were made, they are not an effective cell therapy.
Finally, we come to scalability. We know immuno-oncology works; they are curative procedures, but the current process, due to the nature of being autologous from the vein back to the vein, makes them not very scalable. By making cell therapies more scalable, one could start to think of bringing these therapies to diseases other than terminal diseases.
What is Big Data in the context of cell therapy, and how can it be applied to direct cell conversion?
At Mogrify, we develop data-driven cell therapies. To do this, we integrate large-scale datasets including a wide range of biological information to model cell identity. We are all about defining what makes a cell a cell. But what makes a cell behave this way in this context? To answer this, we leverage extensive cellular profiling with next-generation sequencing in conjunction with other types of biological information such as DNA-protein and protein-protein interactions to build cell- type-specific regulatory networks. This way we can create a platform that allows us to understand and define genetic signatures which help towards identifying a cell and its context. With this information, we can go even further and find key molecular switches for cell identity. Once we understand what defines a cell and its regulatory control points, we can now ask whether we can make it in vitro by converting another cell.
What are the benefits of direct cell conversion without going through an intermediary stage?
These conversions happen in nature but always in one direction: from totipotent to pluripotent, multipotent, bipotent and finally to non-potent. The very first method of cell conversion was discovered by John Gurdon, who won the Nobel Prize in 2012 for his seminal discovery where he noticed that he could reprogram a gut cell back to a pluripotent stage. What his work, and the work of Shinya Yamanaka, on the factors enabling iPSCs, means is that we can convert cells the other way.
Going direct without going through an intermediary stage will enable in vivo conversions. Imagine you want to convert a cell type directly into another using gene therapy. By avoiding the intermediary stage, you can do this conversion in vivo, bypassing potential contamination of the cells with unwanted cell types and saving on time.
What checks or critical quality attributes should manufacturers look for to ensure complete cell conversion has taken place?
With any part of advanced therapeutic manufacturing, there are regimented procedures that need to be followed. Regulatory agencies and manufacturers are learning as we go, but there are six pillars that must always be checked: cell identity, purity, viability, potency, karyology and tumorigenicity.
If you are starting from an iPSC, you must look at the genetic stability of the cell and check if there is a mutational burden. There are routine pluripotent stem markers and pluripotency checks to see if the cells generated are contaminated with pluripotent cells. The most common way is to conduct teratoma assays and karyotypic analysis. If there was genetic engineering involved in transforming those cells, using viral delivery, what is the copy number of the viruses that were used? Did the viral vectors hamper the differentiation process? All this needs to be checked.
Another important attribute is efficacy. One of the main challenges in cell therapy is to understand if the cells are efficacious because cell therapies are so complex. In addition, the only way to understand, for example, if a therapy works to treat Parkinson’s disease, is to engraft it and wait for many years to see if it works. If there was a simple assay that correlates how the cell behaves in the laboratory and how it will behave in the therapy, it would provide criteria for release testing and reduce the cost of developing therapies.
That sounds really complex. How scalable is all of that to commercially manufacture?
Because of this complexity, this has been one of the biggest bottlenecks to scale cell therapy. As scientists, we can complicate things by trying to learn everything; while that is important at the research and development stage, when we come to process development, we need to streamline it. Another difficulty in scalability is the length of time it takes; for example, it takes at least 3 months to make a neuron and for it to show electrophysiological activity.
So, might there be a way that we can scale these processes by reducing the timespan required to make cells in vitro? Here, you need to start thinking of synthetic ways to bypass where natural development is restricting us.
Where do you hope that Mogrify’s platform could be applied in the future?
Considering the bare bones of our platform we believe it constitutes a fundamental and accessible tool for the development of cell therapies and could be applied to pretty any area with a high unmet clinical need. We can look at factors that control aging or look at genetic signatures of healthy cells versus diseased cells. As a company, we are exploring two areas – cellular gene therapy, with in vivo reprogramming, and cell therapy, with in vitro cell conversion – in many therapeutic areas including immunology, ophthalmology and oncology.
Here, the big advantage of converting cells from one state to another is you can do it in vivo, which could fundamentally change how one looks at gene therapy. For example, in the liver, gene therapy has been used to conduct gene replacement. What Mogrify’s platform could allow is the use of gene therapy to deliver factors that control the functioning of the cell to ultimately change the identity of the cell.
What do you think the future of therapeutic cell production might look like?
It is very hard to make predictions, especially about the future! However, I believe we are moving towards a centralized cell production system where, instead of looking at vein-to-vein autologous therapies, we are looking towards a system where cells will be available off-the-shelf in a centralized manner. There may be big banks of, say, iPSCs, all around the world, but how can we get the required tissue? That is where Mogrify and direct cell conversion comes in. Our platform is a chassis, whereas future cell therapies require a whole car. I believe we will start to look at a plug and play systems, where different technology will be centralized to build the final cell therapy product.
If people would like more information, how can they contact you?
You can find me on LinkedIn or email me at [email protected].