James Armstrong on building tissues with ultrasound and 3D printing
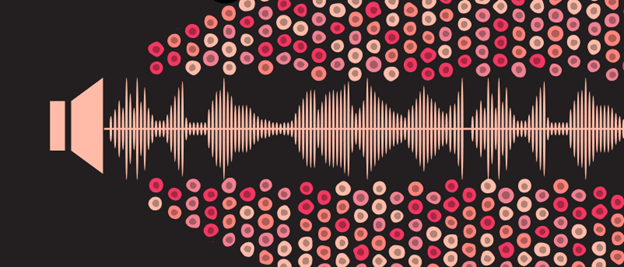
James Armstrong is a senior research fellow based in the Department of Translational Health Sciences at the University of Bristol (UK). In this interview, he discusses his work in producing complex tissue constructs. James sheds light on the techniques that facilitate this goal, such as the use of ultrasound to spatially pattern cells or the 3D bioprinting of vascularized and biomimetic tissues.
Read the full interview below.
Could you give us an insight into the field of tissue engineering and how you are using acoustic cell patterning in this space?
So tissue engineering is the production of artificial living tissues in the laboratory, typically using biomaterials, cells and growth factors. You put these together in different combinations, drawing inspiration from things like wound healing or developmental biology, with the intention to produce a structure that is similar to its native counterpart. The end goal here is to produce clinical tissue grafts or in vitro models, which can be used to understand aspects of disease, development or drug response.
The key thing here for me is the structure of the engineered tissue, something that is often overlooked. If we can produce tissues that have a more faithful structure, then we can replicate some of their higher-order functions. One of the key aspects of structure is the organization of cells and this is where acoustic cell patterning comes in.
Using sound waves, we can remotely manipulate living and unlabeled cells, positioning them in clusters, bands or columns. We can then capture those cells in different biomaterials, then take those patterned biomaterials and run them through a standard course of tissue engineering. This idea really appealed to me because it is non-disruptive — we don’t have to change the whole tissue engineering process, all we need to do is apply a simple sound field at the beginning.
How and why did you gravitate toward acoustic cell patterning?
I have always tried to come at tissue engineering from a slightly different angle. I was a chemist by undergraduate training and my PhD was in functional nanomaterials at the University of Bristol. In my PhD, I was exposed to quite a lot of different techniques from the physical sciences and from engineering; optical tweezers, for example, really fascinated me. Toward the end of my PhD, I encountered this acoustic manipulation approach, which is commonly used by physicists and engineers to manipulate and understand properties of particles.
It struck me that this could be something that could be applied to the life sciences, so I delved into the literature and found out I was not the first person to have this idea! Having said that, I was a very early adopter of such an approach and I think that our work really helped to demonstrate how acoustic cell manipulation can be used for musculoskeletal tissue engineering. I think this process showed me the value of stepping outside of my own discipline and going to talks and seminars that were not immediately relevant, but can actually spark that creative idea or new research direction.
What tissue types have you investigated with this technique?
Initially, in 2018, we published on skeletal muscle. This was the first tissue I looked into and it was the obvious tissue for me because native muscle has really hierarchical, organized structure. You have these bundles of myotubes and fibers, which dictate the mechanical properties of the tissue at the cell level. It was clear to me that if we could organize the cells in muscle tissue, then we could be moving closer to the natural skeletal muscle form. After a lot of work, we successfully developed a device that could pattern myoblasts reproducibly in collagen-based gels. This guided the fusion and differentiation of those myoblasts and created these oriented hierarchical structures. We even saw evidence of mechanical anisotropy in that tissue.
Cartilage was our second publication and was a little bit less intuitive to me because in the body it is the extracellular matrix fibers in cartilage that are important for the mechanical function of the tissue, but we tried it nonetheless. We patterned cells and saw that, over time, they could guide the organization of the surrounding collagen fibers. This for me was a nice advance – not only can we use acoustics to pattern the cells, but then those patterned cell populations can be used to drive the formation of an organized extracellular matrix.
What do you think the future looks like for this technology?
I think we are in a really interesting phase for the technology. People like myself and others have demonstrated pretty conclusively that this technology can be used to organize cells within biomaterials and that these can be used to produce functional structured tissues.
There are definitely technical challenges remaining. The field is striving toward acoustic fields that can be used for more arbitrary (non-geometric) pattern formation, which is challenging, particularly in 3D. There is also the challenge of multicellular patterning, particularly how to differentially pattern two different populations of cells. These technical challenges are starting to be addressed by people like Peer Fisher (Heidelberg University, Germany), Mikhail Shapiro (California Institute of Technology; CA, USA) and Bruce Drinkwater (University of Bristol, UK), who have made some fascinating technological developments. I think as we start to integrate these more advanced manipulation technologies, then that will really drive the concepts through to the next stage.
How are you, or other researchers in the field, driving the uptake of this technique amongst young scientists?
Acoustics is deceptively simple. I am not an acoustician, but with a little bit of help, I was able to generate these devices; my team makes them in the lab all the time. If you have all the components, it is a reasonably simple engineering task but there is definitely a barrier to entry. It is still seen as a niche technology, but I am keen to get it out of my lab and into the hands of scientists across the globe.
In September we had nine people from across the UK and Europe spend three days in my lab for a summer school, learning how to build and operate the acoustic devices. They all took one of the acoustic patterning devices that they made back with them to their labs to explore as they wished. Activities like this are key as well as conducting truly open science by publishing very clear protocols and making them openly accessible to the scientific community.
Another research area of yours is 3D bioprinting. Could you paint a picture of the work you have undertaken in this area?
I mentioned that I am very interested in tissue complexity and how we can organize structures to more closely mimic natural tissues. So acoustic patterning is a very nice technology for organizing cell populations, while bioprinting can be used to structure the material support for those cells and create custom architectures all in a very automated and high throughput manner.
The angle that we are taking with 3D bioprinting is in vascularized tissue engineering. We published some methods previously with a collaborator who is now at Tsinghua University, Liliang Ouyang. We looked at developing methods for producing complex vascular networks throughout different biomaterial systems and we are now looking to adapt this methodology and incorporate different cell types and reproduce vascularized tissues such as bone or cardiac tissue.
Are there any other key developments that are occurring in 3D bioprinting and how might these shape the future of the field?
There are plenty of developments in terms of bioinks. For example, myself and Liliang Ouyang published a method on soft hydrogel printing, which I think is going to be important. Lots of cells and tissues will develop better morphology and produce better extracellular matrix in a soft biomimetic environment, however, it was not previously possible to print tissues at these low stiffnesses. So our advance here was extending the “printability window”, which will open up new opportunities for soft tissue printing.
We are also seeing fantastic advances in things like coaxial bioprinting, multi-axis printing, and in vivo bioprinting. My personal favorite is volumetric bioprinting, which generates large structures in a few seconds using photocrosslinkable gels and projected light, work that is being spearheaded by people like Riccardo Levato (Utrecht University, Netherlands). These kinds of technological developments allow us to create architectures and produce cellular constructs in a way that we were simply not able to 10 years ago. As long as these technologies can be made accessible to the wider community, then we start to see all sorts of creative ways to produce structures that are complex in both biology and architecture.
What challenges are you currently facing in your work and how are you working to address them?
We are always looking at complexity in tissues and in particular how to drive toward multicellular complexity. It is one thing being able to organize a single population of cells in a biomaterial using an acoustic field, but it is a far greater challenge to organize multiple cell types in their relevant configuration and then be able to culture and grow those cells together. To address this you need to tick a lot of boxes – the patterning technology, the biomaterial, the media formulations and how those morphogens and chemical factors are delivered. This is what is keeping my lab occupied right now!
You may also like:
Bioprinting neural tissue: it’s a no-brainer
Bioinks are being used to generate increasingly complex tissue types, as seen in a recently published study that produced replica neural tissue.
The opinions expressed in this interview are those of the author and do not necessarily reflect the views of RegMednet or Taylor & Francis Group.